Microbiome Engineering
Functional Biodiversity
Functional Biodiversity focuses on the technical advances necessary to begin engineering microbiomes based on function in addition to genetics. This shift in approach requires significant advances in functional genomics and microbiome modeling, but will help improve microbiome stability and function over time.
Introduction and Impact
Ultimately, a major goal of microbiome engineering is that these technologies will have functional purposes outside of the lab. Engineered microbiomes out in the world encounter new environmental conditions and different species from those used in the lab. This theme aims to address that technical challenge by examining how microbiomes can be engineered to harness the biodiversity present in these environments and focuses on a species’ function, rather than the specific species present in the microbiome.
Underlying this is the ecological concept of “guilds.” Loosely, guilds are basic structural units of communities and ecosystems. In the context of this roadmap, ecological guilds are a collection of microbes with similar roles/functions within a community, and having similar resource requirements or exploiting resources in similar ways., They can also exist in different niches (while still performing similar functions), resulting in potentially unique interactions with their neighbors compared to other members of the same functional guild. The end result is a holistic view of functional biodiversity – considering the functional diversity of an ecosystem at any level, with an agnostic view of what specific species are in these ecosystems.
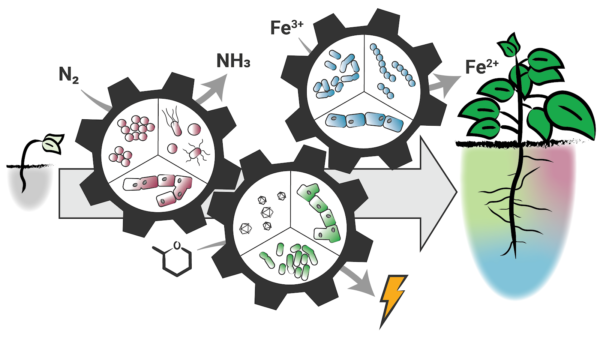
Figure 2. Functional biodiversity in natural and engineered microbiomes. Microbiomes consist of functional guilds – groups of potentially taxonomically distinct organisms that have similar roles in a community – which contribute to the microbiome’s stability and function over time. Guilds (depicted as microbes similarly colored in red, green, and blue) may be genetically unrelated, but can all perform a function, such as nitrogen fixation, energy production, or iron reduction in a soil microbiome. Engineering functional guilds within a microbiome can harness biodiversity such that a task accomplished by any individual guild member (e.g., nitrogen fixation by red fungal cells) is redundant with another guild member (e.g., nitrogen fixation by red cocci) to make the whole community more resilient. Engineering guilds can be accomplished through either top-down (e.g., microbiome enrichment) or bottom-up (e.g., rationally designed consortia) approaches, both of which have unique benefits and drawbacks. Engineering microbiome guilds, rather than individual species, will create stable communities that resist evolutionary pressures.
This is particularly important as technologies are deployed outside controlled laboratory environments, where engineered microbiomes encounter new environmental conditions and microbial species. To maintain functionality, an engineered microbiome must persist in the face of these interactions. Engineering microbiomes based on guilds, rather than individual species, creates a framework in which guild functions can be maintained to ensure robust functionality, without requiring a complete redesign in every new environment.
The first goal of Functional Biodiversity addresses the conceptual shift that will need to occur, in order to design microbiomes based on functional guilds. Current methods for studying microbial communities rely heavily on metagenomics or other sequence-based techniques. This provides information on genetically related organisms, so called “taxonomic guilds,” but developing a function-oriented understanding of microbiomes will require significant advancements in other -omics techniques and approaches for modeling microbial interactions within and between functional guilds.,,,, Subsequent goals focus on designing robust microbial communities based on their function, and then harnessing the flexibility of functional guilds to engineer microbiomes that retain their function as they move from the lab into a natural environment.